Equine bone marrow-derived mesenchymal stem cells: optimization of cell density in primary culture
Introduction
Exciting achievements in the research area of stem cells have brought new hopes of curing the incurable diseases, although the existing methods in this research area have not yet provided good clinical results (1). Until today, adult stem cells have been derived from different human and animal sources, such as bone marrow (BM), peripheral blood, umbilical cord blood, and fat tissue (2,3). The parenchyma of red BM consists of numerous megakaryocytes, plasma cells, monocytes, and hematopoietic stem cells (HSCs), which produce all the three classes of blood cells that exist in the circulatory system (4). The BM stroma consists of fibroblasts, macrophages, adipocytes, osteoblasts, osteoclasts, and endothelial cells, which comprise a special microenvironment and indirectly play a role in hematopoiesis (5).
Another type of cells in the BM is the mesenchymal stem cells (BM-MSCs) (6), which can be used for the treatment of certain diseases. These multipotent stem cells have been differentiated into several specific cells such as osteoblasts, chondrocytes, myoblasts, adipocytes, β-cells of pancreatic islets, and even neurons (7). Due to this ability, MSCs have been successfully used for the treatment of degenerative diseases such as musculoskeletal disorders, including osteoarthritis (8), tendon and ligament injuries (9,10), rheumatoid arthritis (11), spinal cord injury (12,13), liver disease (14), and bone defects (15). Therefore, stem cells, especially MSCs derived from different tissues, are a better promising strategy for curing common orthopedic diseases in horses (16).
Mononuclear cell (MNC) population in the BM consists of a variety of cells such as MSCs, monocytes, lymphocytes, and HSCs (17). MSCs can be easily separated from HSCs through culture and adherence to plastic dishes (18). BM-derived nucleated cells show variations in morphology (19). During sequential subcultures, the heterogeneity decreases and spindle-shaped fibroblast-like cells become predominant (20). The proportion of BM-derived MSCs has been reported to be 1 to 3.8×105 in felines (21), 2.5×104 in canines (22), 104–105 in humans (23), and 1 to 4.2×103 in equines (19).
The number of MSCs obtained through the culture of equine BM samples has been reported to be influenced by the isolation methods (24). Several methods have been used to isolate and culture equine MSCs (24); however, due to the large volume of BM aspirated samples in most of the studies (generally more than 10 ml), the gradient density method is preferred to the classic method (culture of whole BM sample) (24). MNCs have been isolated by the gradient density protocol, except non- or poly-nucleated cells. The presence of these contaminant cells, including erythrocytes, platelets, granulocytes, and myeloid progenitor cells, in the classical method could potentially weaken the proliferation of MSCs (24).
Stanley et al. [1995] have demonstrated the importance of appropriate cell density for the expression of some cell adhesion molecules in the bladder and colonic cell lines. Cell–cell and cell–matrix interactions such as anchorage, migration, proliferation, and differentiation of the cells are being intervened using cell adhesion molecules (25). These interactions could also modify the gene expression and establishment of the cellular scaffold and lead to modification of the morphology of the cells (26). We speculated that attachment of cells to the culture dish surface can be affected by cell density, because it has been shown that the expression of β1 and β4 integrins of adhesion molecules would change with changes in cell density (27). On the other hand, De Schauwer et al. (28) proposed the minimal criteria for defining equine MSCs and demonstrated that β1 integrin (also termed as CD29) is one of their putative surface markers. This implies that β1 integrin is a primary surface marker expressed on MSCs and plays an important role in the adherence and function of these cells. Moreover, Piedimonte et al. (29) demonstrated that the transportation of small nutrients such as amino acids is altered at various cell densities. Another study suggested that this modulation of nutrient transport in normal cells is related to the cell–cell contact that is mediated by adhesion molecules (30).
Considering the above mentioned aspects, it appears that it is necessary to optimize the cell density in the primary culture of bone marrow-derived mononuclear cells (BM-MNCs) for the isolation of MSCs. Therefore, we conducted this study to investigate the effects of MNC number in the primary culture on the yield of MSCs.
Methods
All the media and solutions were purchased from Sigma-Aldrich Company (Germany). The experimental procedure is depicted in Figure 1.
Sample aspiration
BM samples were collected from two healthy 9-year-old mares. After restraining and using ultrasonography to determine the exact sampling place on the sternum, 0.5 mg/kg xylazine was injected intravenously as a sedative. Then, 10 mL of lidocaine (2%) with adrenaline was injected as a local anesthesia around the entrance of the biopsy needle. Using Jamshidi needle (gauge 13, 10-cm length), at least 10 mL of BM was aspirated into a 20-mL syringe containing 10,000 IU heparin (1,000 IU/mL). Within 4 h, all samples were carried on ice to the laboratory for further experiments.
MNC isolation
Samples were thoroughly mixed with twice the volume of basic culture medium (DMEM-high glucose supplemented with 10% FBS, 1% penicillin–streptomycin, and 0.1% amphotericin B) under a sterile laminar hood/cabinet in the laboratory. Two portions of the cell suspension were carefully loaded onto the top of one portion of density gradient media (Histopaque® 1077) in 15-mL polypropylene Falcon tubes. The samples were centrifugated in a swinging bucket rotor at 400 g for 30 min at 4 °C (with the brake off). Then, the layer of MNCs on the top of the density gradient media was collected gently using a Pasteur pipette. These MNCs were rinsed twice with Dulbecco’s phosphate-buffered solution (without Ca2+ or Mg2+) at 600 g for 5 min at 4 °C.
Various seeding densities and culture
The final obtained pellet was resuspended with 4 mL of fresh culture medium, and the cells were counted on a Neubauer hemocytometer counting chamber. Then, cells at different densities (1×105, 2×105, 4×105, 8×105, and 1×106 cells/cm2) were cultured in the usual cell culture flasks (T75 filter-capped flasks, SPL, Korea). These cells, considered as primary (P0), were cultured for 21 days, and their medium was changed every 3 days to eliminate non-adherent or dead cells.
Observation procedures
On the 7th and 14th days after seeding, the colonies containing more than 15 cells (Figure 2) were counted under an inverted microscope (Labomed TCM400 California, USA). On day 21, the approximate percentage of confluency and the exact number of adherent cells were calculated after trypsinization using a Neubauer counting chamber.
Mesenchymal stem cell characterization
The harvested cells at P0 were seeded at the usual density of 5,000 cells/cm2 in new T75 flasks as a first passage (P1). The cell culture was continued until P3, and finally, the P3 cells were subjected to analyses of specific marker expression and in vitro differentiation.
For gene expression analysis, total RNA was isolated using Total RNA Isolation Kit (DENAzist Asia, Iran) according to the manufacturer’s instruction, and cDNA was synthesized using the AccuPower® RT Premix kit (Bioneer, USA). PCR was performed using specific primer sets for analyzing the expressions of CD29 (F: 5'aatcgggacaagttacctca3', R: 5'cttccaaatcagcagcaat3'), CD44 (F: 5'aacctcgggtcccatac3', R: 5'tccattgagcccacttgc3'), CD90 (F: 5'agaataccaccgccaca3', R: 5'ggataagtagaggaccttgatg3'), CD34 (F: 5'tgatgaatcgccgtagt3', R: 5'cgggttgtctcgctga3'), and MHC-II (F: 5'ggaacgggcagcaggacat3', R: 5'aagccattcacagagcagacca3'). GAPDH was used as an internal control (F: 5'tgtcatcaacggaaaggc3', R: 5'gcatcagcagaaggagca3'). Thermal cycling was carried out under the following conditions: initial denaturation at 95 °C for 5 min, followed by 30 cycles of 95 °C for 30 s, 51–61 °C for 45 s, and 72 °C for 1 min. The final elongation was performed at 72 °C for 10 min. The amplified PCR products were electrophoresed with ethidium bromide on a 1.5% agarose gel.
For in vitro differentiation, P3 cells were induced to undergo tri-lineage differentiation. For osteogenic and adipogenic differentiations, 3×105 cells were cultured under defined conditions (29). In addition, 5×105 cells were cultured as a micropellet in a 15-mL Falcon tube for chondrogenic differentiation and were treated as previously described by Alipour et al. (31). In all assays, the control group was cultured with the basic growth medium.
Statistical analysis
Statistical analysis was performed using SPSS statistical software version 17.0 (SPSS Inc., Chicago, IL, USA). Data are expressed as mean ± standard deviation. ANOVA, followed by Tukey’s post hoc test, was conducted to investigate the effects of different cell densities on the colony number, the confluency percentage, and the cell yield. A probability of P<0.05 was considered as statistically significant.
Results
After culturing the BM-derived MNCs at the various densities in P0 (Figure 3) and changing the medium during the culture, the non-adherent and dead cells were discarded. The first colonies were observed at day 4 after seeding. The cells in the BM-derived colonies had a heterogeneous morphology, ranging from star-shaped to triangular and spindle-like cells.
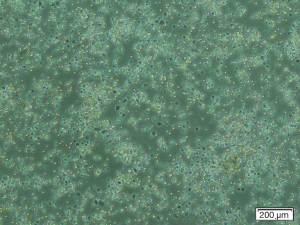
The number of colonies, adherent cells, and the confluency percentage are shown in Table 1. On day 7 after seeding, there was no significant difference in the colony numbers between the groups. Fourteen and 21 days after seeding, the minimum number of colonies, number of adherent cells, and the approximate percentage of confluency were found to be related to the lowest cell density (1×105 cells/cm2), whereas the maximum number of colonies and the approximate confluency percentage were associated with the highest density of cells (1×106 cells/cm2) (P<0.05). However, the maximum number of cells at the end of P0 (day 21), which was utilized for the next subcultures and the expansion of cells, belonged to the intermediate cell density culture (4×105 cells/cm2) (P<0.05). This number was found to be non-significantly higher than that of the group with the highest cell density (1×106 cells/cm2) (P>0.05). Despite the lower number of colonies observed with the intermediate density, these colonies had a large number of cells. We postulated that the cells in these colonies are more dense and exhibit growth overlap (Figure 4). In the culture flasks with the highest cell density, due to the large number of colonies, the cells expanded rapidly and covered a higher percentage of the culture dishes.
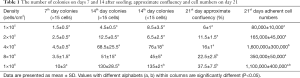
Full table
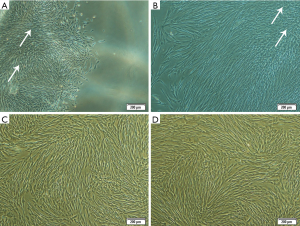
Cells at P3 expressed CD29, CD44, and CD90 surface marker, but not CD34 (hematopoietic progenitor cell marker) and MHC-II (Figure 5). Moreover, the cells differentiated into osteogenic, adipogenic, and chondrogenic lineages (Figure 6).

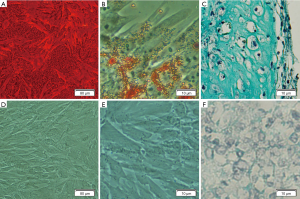
Discussion
Based on our findings, it can be concluded that a high primary cell density does not have a negative impact on the adherence of MSCs to the flasks, although the intermediate seeding density of MNCs (4×105) resulted in more numbers of MSCs at the end of P0 (Table 1). Obtaining high enough cell number at the end of P0 is very important, because at the next passage (P1), more flasks can be subcultured with a proper density (3×103 cells/cm2), and finally, sufficient cell numbers can be achieved at P3 or P4. In morphology assessment, heterogeneous cell population was observed which is similar to that reported by Vidal et al. (19), however, Giovannini et al. (32) reported that the fibroblastic morphology of all the BM-derived MSCs was similar throughout different passages. Increasing the passage number results in the homogeneity of cells (19), which can be used for further analysis or for treatment purposes.
Despite the lower confluency in the flasks seeded with 4×105 cells/cm2, these flasks had a greater number of cells in comparison with the higher ones (8×105 and 1×106 cells/cm2) because of the more dense colonies and the proliferation of cells in an overlapping pattern or almost as a bilayer. As reported by Stanley et al. [1995] indicating that low and excessive cell density leads to a decrease in expression of β1 and β4 integrins, it is probable that the density of 4×105 cells/cm2 would result in an optimum expression of these adhesion molecules and, consequently to an optimum cell–cell and cell–matrix interaction (25). Nonetheless, it is possible to isolate MSCs from a lower cell density such as 1×105 cells/cm2, which is applicable in cases where only a small amount of aspirated BM is available.
As the minimal criteria of equine mesenchymal stem cells (28), cultured P3 cells expressed the related specific genes (CD29, CD44, and CD90) and had trilineage differentiation potential which confirm the isolated cells are equine MSCs. Because of the lack of equine specific antibodies and/or few or no cross-reactivity with other species antibodies (33), detection of markers’ expression at mRNA level by reverse transcription-PCR in equine MSCs would be a good technique that similarly replicates the findings of flow-cytometry technique (34).
Conclusions
Densities of 1×106 and, especially, 4×105 cells/cm2 are recommended for the isolation of equine MSCs based on the amount and volume of BM sample. In addition, regarding large cell numbers, which are required for experiment or treatment, the initial density of 4×105 is suggestible to reach the adequate cell numbers at the end of P0. Finally, these homogenized MSCs can be used for both research and clinical purposes.
Acknowledgements
The authors gratefully acknowledge Azaraksh Sport Club, Mashhad, Iran, for their cooperation for collection of bone marrow samples from horses. We acknowledge the Ferdowsi University of Mashhad for financial support.
Footnote
Conflicts of Interest: The authors declare that there is no conflict of interest.
Ethical Statement: The animal protocol of experiment was approved by The Ethics Committee and Animal Welfare of Ferdowsi University of Mashhad.
References
- Ribitsch I, Burk J, Delling U, et al. Basic science and clinical application of stem cells in veterinary medicine. Bioreactor Systems for Tissue Engineering II: Springer; 2010:219-63.
- Sakaguchi Y, Sekiya I, Yagishita K, et al. Comparison of human stem cells derived from various mesenchymal tissues: Superiority of synovium as a cell source. Arthritis Rheum 2005;52:2521-9. [Crossref] [PubMed]
- Tondreau T, Meuleman N, Delforge A, et al. Mesenchymal Stem Cells Derived from CD133-Positive Cells in Mobilized Peripheral Blood and Cord Blood: Proliferation, Oct4 Expression, and Plasticity. Stem Cells 2005;23:1105-12. [Crossref] [PubMed]
- Wintrobe MM, Greer JP. Wintrobe's clinical hematology. Philadelphia: Lippincott Williams & Wilkins; 2009.
- Thiriet M. Hematopoiesis. Tissue Functioning and Remodeling in the Circulatory and Ventilatory Systems. Biomathematical and Biomechanical Modeling of the Circulatory and Ventilatory Systems. 5: Springer New York; 2013:19-52.
- Dominici M, Le Blanc K, Mueller I, et al. Minimal criteria for defining multipotent mesenchymal stromal cells. The International Society for Cellular Therapy position statement. Cytotherapy 2006;8:315-7. [Crossref] [PubMed]
- Lakshmipathy U, Verfaillie C. Stem cell plasticity. Blood Rev 2005;19:29-38. [Crossref] [PubMed]
- Litzke LE, Wagner E, Baumgaertner W, et al. Repair of extensive articular cartilage defects in horses by autologous chondrocyte transplantation. Ann Biomed Eng 2004;32:57-69. [Crossref] [PubMed]
- Pacini S, Spinabella S, Trombi L, et al. Suspension of bone marrow-derived undifferentiated mesenchymal stromal cells for repair of superficial digital flexor tendon in race horses. Tissue Eng 2007;13:2949-55. [Crossref] [PubMed]
- Smith RK. Mesenchymal stem cell therapy for equine tendinopathy. Disabil Rehabil 2008;30:1752-8. [Crossref] [PubMed]
- Augello A, Tasso R, Negrini SM, et al. Cell therapy using allogeneic bone marrow mesenchymal stem cells prevents tissue damage in collagen-induced arthritis. Arthritis Rheum 2007;56:1175-86. [Crossref] [PubMed]
- Adel N, Gabr H. editors. Stem cell therapy of acute spinal cord injury in dogs. Third World Congress of Renerative Medicine Regen Med; 2007: Future Medicine.
- Chopp M, Zhang XH, Li Y, et al. Spinal cord injury in rat: treatment with bone marrow stromal cell transplantation. Neuroreport 2000;11:3001-5. [Crossref] [PubMed]
- Kallis YN, Alison MR, Forbes SJ. Bone marrow stem cells and liver disease. Gut 2007;56:716-24. [Crossref] [PubMed]
- Kraus KH, Kirker-Head C. Mesenchymal stem cells and bone regeneration. Vet Surg 2006;35:232-42. [Crossref] [PubMed]
- Borjesson DL, Peroni JF. The regenerative medicine laboratory: facilitating stem cell therapy for equine disease. Clin lab Med 2011;31:109-23. [Crossref] [PubMed]
- Michler RE. Stem Cell Therapy for Heart Failure. Methodist Debakey Cardiovasc J 2013;9:187-94. [Crossref] [PubMed]
- Friedenstein AJ, Chailakhjan RK, Lalykina KS. The development of fibroblast colonies in monolayer cultures of guinea-pig bone marrow and spleen cells. Cell Tissue Kinet 1970;3:393-403. [Crossref] [PubMed]
- Vidal MA, Kilroy GE, Johnson JR, et al. Cell growth characteristics and differentiation frequency of adherent equine bone marrow–derived mesenchymal stromal cells: adipogenic and osteogenic capacity. Vet Surg 2006;35:601-10. [Crossref] [PubMed]
- Kulterer B, Friedl G, Jandrositz A, et al. Gene expression profiling of human mesenchymal stem cells derived from bone marrow during expansion and osteoblast differentiation. BMC Genomics 2007;8:70. [Crossref] [PubMed]
- Martin DR, Cox NR, Hathcock TL, et al. Isolation and characterization of multipotential mesenchymal stem cells from feline bone marrow. Exp Hematol 2002;30:879-86. [Crossref] [PubMed]
- Kadiyala S, Young R, Thiede M, et al. Culture expanded canine mesenchymal stem cells possess osteochondrogenic potential in vivo and in vitro. Cell Transplant 1997;6:125-34. [Crossref] [PubMed]
- Pittenger MF, Mackay AM, Beck SC, et al. Multilineage potential of adult human mesenchymal stem cells. Science 1999;284:143-7. [Crossref] [PubMed]
- Bourzac C, Smith LC, Vincent P, et al. Isolation of equine bone marrow-derived mesenchymal stem cells: a comparison between three protocols. Equine Vet J 2010;42:519-27. [Crossref] [PubMed]
- Stanley AJ, Banks RE, Southgate J, et al. Effect of cell density on the expression of adhesion molecules and modulation by cytokines. Cytometry 1995;21:338-43. [Crossref] [PubMed]
- Ben-Ze'ev A, Robinson GS, Bucher N, et al. Cell-cell and cell-matrix interactions differentially regulate the expression of hepatic and cytoskeletal genes in primary cultures of rat hepatocytes. Proc Natl Acad Sci U S A 1988;85:2161-5. [Crossref] [PubMed]
- Albelda SM, Buck CA. Integrins and other cell adhesion molecules. FASEB J 1990;4:2868-80. [Crossref] [PubMed]
- De Schauwer C, Meyer E, Van de Walle GR, et al. Markers of stemness in equine mesenchymal stem cells: a plea for uniformity. Theriogenology 2011;75:1431-43. [Crossref] [PubMed]
- Piedimonte G, Borghetti AF, Guidotti GG. Effect of cell density on growth rate and amino acid transport in simian virus 40-transformed 3T3 cells. Cancer Res 1982;42:4690-3. [PubMed]
- Pardee AB. The cell surface and fibroblast proliferation some current research trends. Biochim Biophys Acta 1975;417:153-72. [PubMed]
- Alipour F, Parham A, Kazemi Mehrjerdi H, et al. Equine Adipose-Derived Mesenchymal Stem Cells: Phenotype and Growth Characteristics, Gene Expression Profile and Differentiation Potentials. Cell J 2015;16:456-65. [PubMed]
- Giovannini S, Brehm W, Mainil-Varlet P, et al. Multilineage differentiation potential of equine blood-derived fibroblast-like cells. Differentiation 2008;76:118-29. [Crossref] [PubMed]
- Ibrahim S, Steinbach F. Non-HLDA8 animal homologue section anti-leukocyte mAbs tested for reactivity with equine leukocytes. Vet Immunol Immunopathol 2007;119:81-91. [Crossref] [PubMed]
- Radcliffe CH, Flaminio MJ, Fortier LA. Temporal analysis of equine bone marrow aspirate during establishment of putative mesenchymal progenitor cell populations. Stem Cells Dev 2010;19:269-82. [Crossref] [PubMed]
Cite this article as: Zahedi M, Parham A, Dehghani H, Kazemi Mehrjerdi H. Equine bone marrow-derived mesenchymal stem cells: optimization of cell density in primary culture. Stem Cell Investig 2018;5:31.