Cell sources for regenerative medicine of the liver and endoderm organs: strategies and perspectives
In a recent paper, Wang and colleagues showed that a set of defined small molecules plus mesenchymal feeder cells can covert human gastric epithelial cells into induced endodermal progenitors with the capability to differentiate into liver and pancreatic adult parenchymal cells (1). This manuscript could open new perspective in the field of cell therapies for endodermal organs suggesting the stomach as a possible new cell source for the support of liver and pancreatic functions.
In the field of liver diseases, orthotopic liver transplantation (OLT) represents the only curative treatment for acute liver failure and end-stage chronic liver disease (2). However, OLT is limited by severe shortage of organ donors and nearly 15% of patients die on the waiting list (3,4). Therefore, the development of cell therapy strategies represents an interesting curative approach and could represent a possible option to support liver function in the waiting of transplantation.
Cell therapies with mature hepatocytes have provided encouraging results in several clinical trials and adult hepatocyte transplantation has been indicated as a promising alternative to OLT for the treatment of some liver-based metabolic disorders or acute liver failure (5); unfortunately, the effects of hepatocyte transplantation are transient and cell function appears to decline after few months; moreover, cell transplantation procedures cause complications and require immunosuppression (5). Actually, one of the main obstacles to the clinical application of hepatocyte transplantation resides in sourcing of cells and in difficulties in cryopreservation, and in low engraftment (5). Isolation of primary human hepatocytes competes with organs for OLT; therefore, current sources of tissue for hepatocyte isolation are limited and of marginal quality, such as adult organs that are unsuitable for OLT, livers that have undergone aggressive therapies, or surrounding liver parenchyma obtained after tumor excision (5). As regard, cryopreserved cells, absence of proliferation and reduced functions of thawed hepatocytes damper their potential for cell therapies (5).
The improvement of regenerative medicine approaches for liver diseases requires the identification of sustainable and readily available cell sources (2). In keeping, the possibility of reprogramming adult somatic cells attracted attention for the possibility to generate mature hepatocyte readily available for transplantation (4). In this field, numerous advances have been obtained since the reprogramming of human fibroblasts into induced pluripotent stem cells (iPSC) using a retroviral vector by Yamanaka and associates (6); up to date, human iPSC lines can be generated not only using fibroblasts cultured from a skin biopsy but additional cell types have been proved to be susceptible to reprogramming such as keratinocytes, blood cells, and mesenchymal stem cells (MSC) (4). In parallel, significant advancements have been obtained in the definition of protocols for the differentiation of human iPSC into functional mature hepatocytes (iPSC-Hep). Interestingly, all the published protocols recapitulate the major developmental steps leading to liver organogenesis with initial differentiation of iPSCs into definitive endoderm, then hepatic specified endoderm, followed by the formation of hepatic progenitors and finally differentiation into functional hepatocyte-like cells (7).
A step forward has been made by the demonstration that reprogramming strategies do not require the establishment of stable iPSC lines but mature hepatocytes (iMPC-Heps: induced multipotent progenitor cell-derived hepatocytes) can be obtained after partial reprogramming to pluripotency followed by directed hepatocyte differentiation (8). More recently, induced hepatocytes (iHeps) have been directly reprogrammed from fibroblasts (or MSCs) by forcing the expression of hepatic transcription factors (6); Huang et al. were able to generate iHeps using three hepatic transcription factors (9); similarly, Du et al. generated functional iHeps from fibroblasts using six hepatic transcription factors in combination with MYC overexpression and TP53 knockdown (10).
The clinical application of reprogrammed cells raises concerns about their actual use and the open possibility that these cells could undergo reversion to more primitive state with uncontrolled tumorigenic expansion within the recipient (2). In particular, a critical point for the translation in clinics resides in the frequent occurrence of chromosomal and subchromosomal abnormalities in reprogrammed cells (4). In addition, genomic abnormalities have been reported characterized by the frequent presence of regions with copy number variations of various sizes, either deletions or duplications (4). Moreover, the number of mutations in a human iPSC line is not stable and long term culture may impact the genomic integrity of the cells (2,4). In addition to genomic abnormalities, it is well described that reprogramming is also associated with epigenetic changes (11). These aspects could be particularly crucial for pediatric liver diseases in the light of long life expectancy and in chronic liver diseases since these conditions were characterized by an “adverse niche” characterized by a chronic inflammatory milieu which can favor tumorigenesis (2).
The optimal reprogramming method with no impact on the cell genome is not yet established and reprogrammed cells lack sufficient maturity for widespread clinical use (2,5). In keeping, the small molecule-based reprogramming has attracted much interest due to its safety and efficiency in controlling cell fates (12); this methodology has been successfully used for fibroblast reprogramming into iPS or neurons (13). In this context, the recent paper by Wang et al. described a novel approach to generate human induced endodermal progenitor cells (hiEndoPCs); the lineage reprogramming of gastrointestinal epithelial cells was obtained using a cocktail of defined small molecules in association with the support of tissue-specific mesenchymal feeders (1). In terms of transcriptional and epigenetic signatures, hiEndoPCs have been proved to be a particular stage of endodermal progenitor cells which is intermediate between primitive gut-tube and posterior foregut (1). In vitro, hiEndoPCs clonally expand in culture and have multipotent capability when treated with defined soluble molecules; to this latter regard, hiEndoPCs can give rise to hepatocytes, pancreatic endocrine cells, and intestinal epithelial cells. Interestingly, the authors showed that hiEndoPC-derived hepatic cells are fully functional by testing in vitro properties and in vivo capability to rescue liver functions in a murine model of tyrosinemia type I liver disease. Importantly, hiEndoPC maintained a normal karyotype during five continuous passages in vitro and showed no tumors formation over 6 months after subcutaneous transplantation in mice, thus suggesting minimal tumorigenic potential capacity if compared to iPSC or embryonic stem cells (1). Since human gastric epithelial cells are readily available from human donors of many ages, the conversion strategy proposed by Wang and associates can generate clonally expandable cell populations with a variety of potential applications, including disease modeling and personalized drug screening; moreover, their low tumorigenic potentiality makes them more acceptable for future clinical applications. However, extensive pre-clinical studies in experimental models are needed before reprogrammed cells and their derivatives can be used in human therapy. This is mostly due to the fact that all these cells need several in vitro manipulations to generate mature cells; thus, safety issues and the definition of actual protocols should by strictly addressed to meet criteria for translation into clinical programs (2,3).
Besides cell reprogramming, the use of MSCs or determined stem/progenitor cells isolated from adult or fetal human organs has been proposed for the regenerative medicine of the liver (3). Adult or fetal stem cells have the advantage to require only minimal manipulation with respect to reprogrammed cells. In keeping, MSCs are easily sourced, readily cryopreserved, and involve transplantation procedures with minimal, if any, complications (3). Therefore, several clinical trials based on the use of MSCs in the treatment of liver diseases have been performed (3). The obtained results clearly indicated that the therapy with MSCs in patients affected by liver diseases offers only short-term effects consisting in alleviation of disease symptoms; inefficient lineage restriction to mature hepatocytes has been observed in clinical trials and confirmed in experimental models; actually, the beneficial effects of MSC transplantation have been ascribed to immuno-modulatory properties and paracrine signaling mechanisms (3).
On the other hand, determined stem/progenitor cell population resides into the adult and fetal human liver; in adults, hepatic stem/progenitor cells (HpSCs) are located within the canals of Hering and bile ductules; HpSCs are facultative stem cells characterized by an unique phenotype and able to differentiate into functional mature hepatocytes and cholangiocytes (14). In fetal liver, HpSCs form the ductal plate and give rise to the generation of cholangiocytes of interlobular bile ducts and to periportal hepatocytes during liver development (14). HpSCs can be easily isolate from human livers on the basis of the expression of specific surface antigens such as Epithelial Cell Adhesion Molecule (EpCAM) (15). Moreover, a single Leucine-rich repeat-containing G-protein coupled receptor 5 (Lgr5)-positive liver stem cell can be expanded to form epithelial spheroids in vitro and was able to differentiate into functional hepatocytes in vitro and in vivo (14,16). Organoids containing Lgr5+ cells were able to be expanded ex vivo and to give rise to hepatocytes and cholangiocytes (16). Long-term expansion of adult bile duct-derived bipotent progenitor cells has been obtained from human liver (17,18). A key feature of the expanded cells is the high stability at the chromosome and structural level and the single base changes occur at very low rates (16). Expanded cells can readily be converted into functional hepatocytes in vitro and upon transplantation in vivo (16). Therefore, these properties indicated HpSCs isolated from adult or fetal human livers as possible source for cell therapy program. However, few clinical trials were started with the use of human HpSCs. To date, the only completed trial is the one under the management of Dr. Chittoor Habibullah and his associates at the Liver Institute in Hyderabad (India) (19). This trial used as cell source human HpSCs and hepatoblasts isolated by EpCAM sorting from fetal livers. These investigators focused on patients affected by liver diseases of different etiologies such as biliary atresia, inborn errors of metabolism (Crigler-Najjar), non-alcoholic steatohepatitis, viral cirrhosis, alcoholic cirrhosis and drug toxicity. Results obtained by Habibullah and coworkers suggest that human HpSCs can be effective in treating patients with liver disease. A representative early publication concerned a trial of 25 subjects and 25 controls with decompensated liver cirrhosis due to various causes; subjects received fetal liver-derived EpCAM+ cell infusions into the liver via the hepatic artery. At a 6-month follow-up, multiple diagnostic and biochemical parameters showed clear improvement, and there was a significant decrease in the patients’ MELD scores (19). In western countries, an analogue clinical trial using cells isolated from fetal livers was started (20). Remarkably, immune suppression was not required, although donors and recipients were not matched for histocompatibility antigens. This could be due to the intrinsic immunomodulatory properties of liver stem cells (21).
Another possible source for stem/progenitor cells for liver regenerative medicine is represented by the extrahepatic biliary tree, including the gallbladder and the hepato-pancreatic ampulla (22,23). A key advantage is their ready availability from adult or fetal donors and from cholecystectomized patients in comparison with the scarcity of marginal livers usable for the isolation of adult HpSCs. Extrahepatic biliary tree contains a large population of progenitor/stem cells named biliary tree stem/progenitor cells (BTSCs) (17). From an anatomical point of view, BTSCs reside within peribiliary glands (17); embryological, they represent the remnant in the adult organs of the common biliopancreatic progenitors derived from the ventral endoderm of primitive duodenum (14). BTSCs can be easily isolated from adult or fetal organs, rapidly and clonal expand in culture and can differentiate toward several endoderm cell types including hepatocytes, cholangiocytes and pancreatic endocrine cells (17,24). A preliminary clinical report indicated the feasibility and safety of BTSC transplantation into the liver of patients with end-stage chronic liver disease (20). In general, the use of determined stem cells has the advantage of lower manipulation in comparison with reprogrammed cells and no need of immunosuppression in comparison with mature cells.
In conclusion, the development of novel strategies and the identification of novel cell sources should represent main efforts in the field of cell therapy for endodermal organs (Figure 1). Cell reprogramming strategies are optimal for establishing a stable cell source but safety issues should be addressed prior to translation in clinics. In this context, the study by Wang et al. strongly suggests that a preferable cell sources for reprogramming would be represented by organs with an endodermal origin (1); this approach would simplify protocols and reduce genetic manipulation; moreover, differentiation of reprogrammed cells towards mature endoderm cells would be facilitated since reprogrammed cells partly maintain an epigenetic memory of the starting cell type, leading to a preferential differentiation potential into the cell types they are derived from (25). On the other side, stem cell strategies should take in account the organ availability from donor organs or surgical procedures. However, the presence, phenotype and potentiality of a specific progenitor/stem cell population in adult organs are strongly associated with anatomical features and embryological development. Therefore, stem cells obtained from organs with an endodermal origin would be more prone to differentiate into mature endodermal cells once transplanted and would be more adapt to clinical translation if compared with stem cells with a different embryological origin, such as MSCs.
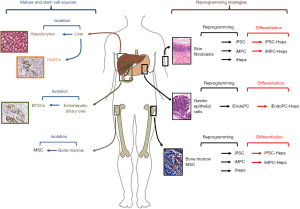
Acknowledgements
Funding: This work was supported by Sapienza University of Rome to E Gaudio; and Ministero dell’Istruzione, Università e Ricerca (FIRB # RBAP10Z7FS_001 to E Gaudio, PRIN # 2009X84L84_001 to E Gaudio).
Footnote
Conflicts of Interest: The authors have no conflicts of interest to declare.
References
- Wang Y, Qin J, Wang S, et al. Conversion of Human Gastric Epithelial Cells to Multipotent Endodermal Progenitors using Defined Small Molecules. Cell Stem Cell 2016;19:449-61. [Crossref] [PubMed]
- Forbes SJ, Gupta S, Dhawan A. Cell therapy for liver disease: From liver transplantation to cell factory. J Hepatol 2015;62:S157-69. [Crossref] [PubMed]
- Lanzoni G, Oikawa T, Wang Y, et al. Concise review: clinical programs of stem cell therapies for liver and pancreas. Stem Cells 2013;31:2047-60. [Crossref] [PubMed]
- Hannoun Z, Steichen C, Dianat N, et al. The potential of induced pluripotent stem cell derived hepatocytes. J Hepatol 2016;65:182-99. [Crossref] [PubMed]
- Dhawan A. Clinical human hepatocyte transplantation: Current status and challenges. Liver Transpl 2015;21 Suppl 1:S39-44. [Crossref] [PubMed]
- Rezvani M, Grimm AA, Willenbring H. Assessing the therapeutic potential of lab-made hepatocytes. Hepatology 2016;64:287-94. [Crossref] [PubMed]
- Forbes SJ, Newsome PN. New horizons for stem cell therapy in liver disease. J Hepatol 2012;56:496-9. [Crossref] [PubMed]
- Zhu S, Rezvani M, Harbell J, et al. Mouse liver repopulation with hepatocytes generated from human fibroblasts. Nature 2014;508:93-7. [Crossref] [PubMed]
- Huang P, Zhang L, Gao Y, et al. Direct reprogramming of human fibroblasts to functional and expandable hepatocytes. Cell Stem Cell 2014;14:370-84. [Crossref] [PubMed]
- Du Y, Wang J, Jia J, et al. Human hepatocytes with drug metabolic function induced from fibroblasts by lineage reprogramming. Cell Stem Cell 2014;14:394-403. [Crossref] [PubMed]
- Liang G, Zhang Y. Genetic and epigenetic variations in iPSCs: potential causes and implications for application. Cell Stem Cell 2013;13:149-59. [Crossref] [PubMed]
- Zhu S, Li W, Zhou H, et al. Reprogramming of human primary somatic cells by OCT4 and chemical compounds. Cell Stem Cell 2010;7:651-5. [Crossref] [PubMed]
- Hu W, Qiu B, Guan W, et al. Direct Conversion of Normal and Alzheimer's Disease Human Fibroblasts into Neuronal Cells by Small Molecules. Cell Stem Cell 2015;17:204-12. [Crossref] [PubMed]
- Lanzoni G, Cardinale V, Carpino G. The hepatic, biliary, and pancreatic network of stem/progenitor cell niches in humans: A new reference frame for disease and regeneration. Hepatology 2016;64:277-86. [Crossref] [PubMed]
- Schmelzer E, Zhang L, Bruce A, et al. Human hepatic stem cells from fetal and postnatal donors. J Exp Med 2007;204:1973-87. [Crossref] [PubMed]
- Huch M, Gehart H, van Boxtel R, et al. Long-term culture of genome-stable bipotent stem cells from adult human liver. Cell 2015;160:299-312. [Crossref] [PubMed]
- Cardinale V, Wang Y, Carpino G, et al. Multipotent stem/progenitor cells in human biliary tree give rise to hepatocytes, cholangiocytes, and pancreatic islets. Hepatology 2011;54:2159-72. [Crossref] [PubMed]
- Wang Y, Lanzoni G, Carpino G, et al. Biliary tree stem cells, precursors to pancreatic committed progenitors: evidence for possible life-long pancreatic organogenesis. Stem Cells 2013;31:1966-79. [Crossref] [PubMed]
- Khan AA, Shaik MV, Parveen N, et al. Human fetal liver-derived stem cell transplantation as supportive modality in the management of end-stage decompensated liver cirrhosis. Cell Transplant 2010;19:409-18. [PubMed]
- Cardinale V, Carpino G, Gentile R, et al. Transplantation of human fetal biliary tree stem/progenitor cells into two patients with advanced liver cirrhosis. BMC Gastroenterol 2014;14:204. [Crossref] [PubMed]
- Riccio M, Carnevale G, Cardinale V, et al. The Fas/Fas ligand apoptosis pathway underlies immunomodulatory properties of human biliary tree stem/progenitor cells. J Hepatol 2014;61:1097-105. [Crossref] [PubMed]
- Cardinale V, Wang Y, Carpino G, et al. The biliary tree--a reservoir of multipotent stem cells. Nat Rev Gastroenterol Hepatol 2012;9:231-40. [Crossref] [PubMed]
- Carpino G, Cardinale V, Gentile R, et al. Evidence for multipotent endodermal stem/progenitor cell populations in human gallbladder. J Hepatol 2014;60:1194-202. [Crossref] [PubMed]
- Carpino G, Puca R, Cardinale V, et al. Peribiliary Glands as a Niche of Extrapancreatic Precursors Yielding Insulin-Producing Cells in Experimental and Human Diabetes. Stem Cells 2016;34:1332-42. [Crossref] [PubMed]
- Kim K, Zhao R, Doi A, et al. Donor cell type can influence the epigenome and differentiation potential of human induced pluripotent stem cells. Nat Biotechnol 2011;29:1117-9. [Crossref] [PubMed]
Cite this article as: Carpino G, Gaudio E. Cell sources for regenerative medicine of the liver and endoderm organs: strategies and perspectives. Stem Cell Investig 2016;3:91.